Matter is frequently divided into three different states, namely solid, liquid and gaseous state. These three states differ in the order and mobility of molecules and rely on the interplay of non-covalent forces and thermal movement. In the solid state, the molecules are close together and their position is fixed by intermolecular forces. By heating substances they usually start to melt at a specific temperature and a phase transitions occurs (from solid to liquid). The molecules of a liquid are still close to each other, however they are able to move around and lose most of their order. Further heating finally leads to evaporation and a gas is formed with molecules moving completely independent from each other.
1888, Friedrich Reinitzer described the unusual behavior of cholesteryl benzoate showing an additional phase of matter occurring between the solid and the liquid state – the liquid crystalline state. Liquid crystals combine the order of crystals with the mobility of liquids and since they occur between these two states they are also called mesogens.
Ordinary fluids are isotropic in nature, meaning their physical properties (e.g. optical, electrical or magnetical) appear the same from any direction in space. Molecules highly anisotropic in shape might exhibit liquid crystalline properties leading to unusual and technologically relevant behavior due to their anisotropy. Many compounds show liquid crystalline behavior including polymers, micelles or biological macromolecules such as DNA or the tobacco mosaic virus. However, the focus of the following discussion will be on low molecular weight liquid crystals.
Liquid crystals are commonly classified by their behavior into thermotropic and lyotropic liquid crystals. Thermotropic liquid crystals show phase transitions in the bulk material upon heating. Thereby, the liquid crystal transfers from its crystalline state (positional and orientational order) to its mesophase (only orientational order) and finally turns into an isotropic liquid (no order). If the mesophases occurs upon heating and cooling it is termed enantiotropic. However, commonly mesophases are fairly narrow and might appear only during cooling or heating (monotropic mesophases). The phase transitions of lyotropic liquid crystals additionally depend on the concentration of the mesogen in the present solvent.
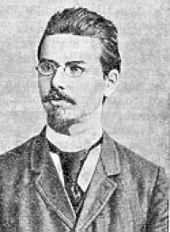
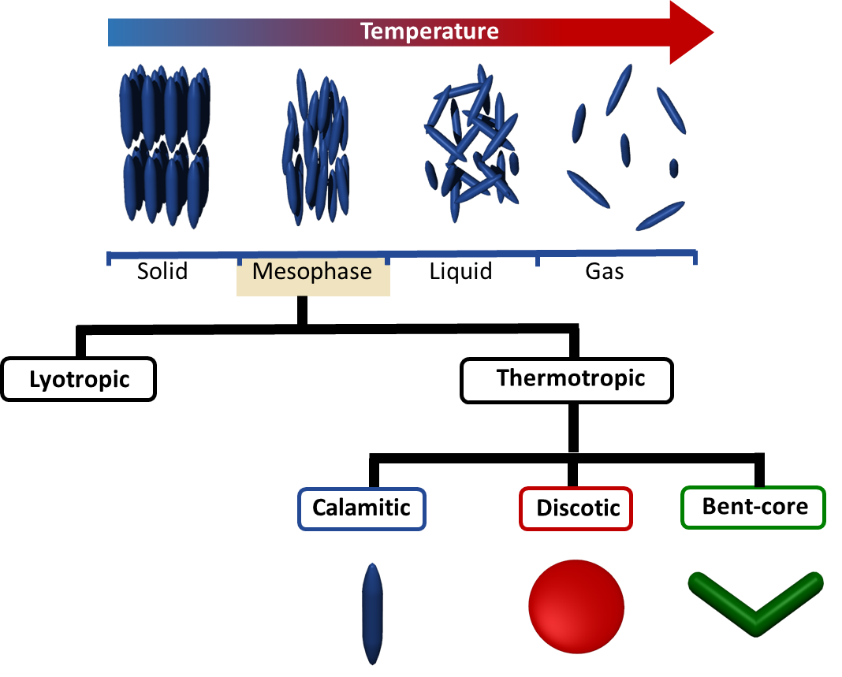
Another common distinction of thermotropic mesogens is with respect to the molecular shape: calamitic (rod-like), discotic (disk-like) and bent-core liquid crystals (also referred to as banana liquid crystals). The molecular design of thermotropic liquid crystals is generally similar. A rigid part (usually derived from arenes) is attached to one or more flexible chains (e.g. alkyl chains) and a polar group. Thus anisotropy in shape allows preferential alignment of the mesogen along one spatial direction.
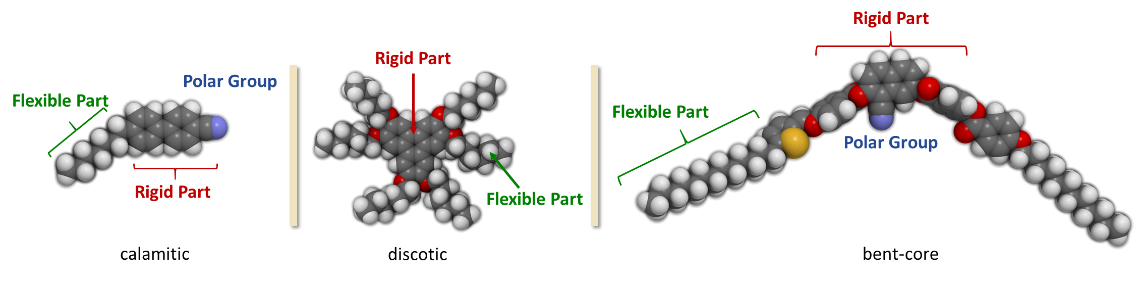
Discotic liquid crystals are flat molecules consisting of a rigid core with flexible side chains in its periphery as shown above. The disk-like shape enables two-dimensional ordering into columnar phases. Discotic mesogens were discovered in 1977 by Chandrasekhar and Billard.
2. Liquid Crystal Mesophases
Liquid crystals show a number of mesophases depending on their molecular shape and nature. The various mesophases are characterized by the degree of order, which is extensive on the molecular scale and often extends up to entire domains of micrometer size. Usually this domains do not extend up to the macroscopic level. However, by applying magnetic or electric fields the order of the single domains is enforced up the macroscopic scale. The most common mesophases of calamitic liquid crystals are the nematic and the smectic phase, while discotic mesogens show columnar and nematic phases.
The nematic phase (N) is one of the most common and by far the most important mesophases for applications. The term nematic refers to the Greek word nema, meaning "thread" and originates from the thread-like topological defects observed in nematics. The nematic phase is the least ordered mesophases of liquid crystals and has only long-range orientational order (no positional order). The molecules are arranged on the average parallel to each other, defining the so-called “director”. It should be noticed that liquid crystals show fluidity and although an average order can be defined, the molecules are constantly flowing and changing position and order. By applying external magnetic or electrical fields the nematics can be easily aligned, which makes them useful for applications such as in liquid crystal displays (LCD). Chiral mesogens show chiral nematic (N*, asterisk denotes the chiral phase) or cholesteric phase. The term cholesteric phase refers back to the first chiral nematic liquid crystals, which was a cholesterol derivative. The molecules of the chiral nematic phase are twisting perpendicular to the director, with the molecular axis parallel to the director. The twist angle between adjacent molecules results from the asymmetric packing leading to the longer-range chiral order. The distance along the helical pitch for a complete rotation of the mesogens is called the helical pitch and is a strong function of temperature.
Smectic phases (Sm) are usually found at lower temperatures than the nematic and show orientational and some degree of positional order. The molecules in a smectic phase are alligned in layers perpendicular to the director. The word "smectic" originates from the Latin word "smecticus" and refers to the soap like properties of smectic liquid crystals caused by the sliding layers. There are several smectic phases known, which differ in the degree of positional and orientational order. Two of the most common ones are the smectic A phase (molecules are oriented along the layer normal) or the smectic C phase (molecules are tilted away from the layer normal). These phases are liquid-like within the layers. There are many different smectic phases, all characterized by different types and degrees of positional and orientational order. Chiral mesogens may exhibit the smectic C* phase. The molecules aligned in layers (as in other smectic phases) tilting by a finite angle with respect to the layer normal leading to an azimuthal twist from one layer to the next.
Discotic mesogens show nematic (with the disks aligned parallel to each other) and columnar phases. The columns themselves may be organized into hexagonal or rectangular arrays. Chiral discotic phases are also known and show a twist of molecules within the columnar arrangement.
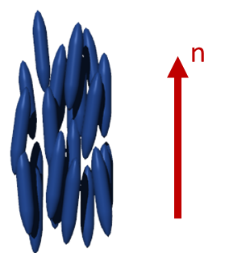
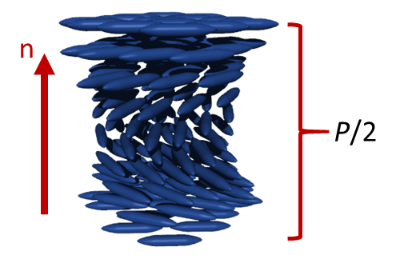
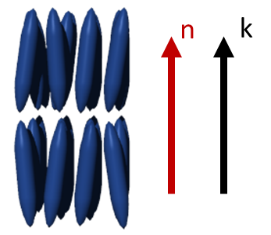
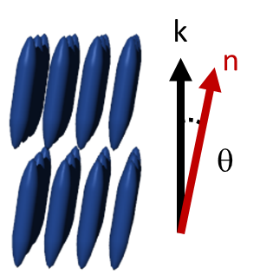
3. Properities of Liquid Crystals
The anisoptropy in the order of liquid crystals results in different electrical and optical parameters if considered along the director or in a plane perpendicular to it. Thus molecules in their mesophases show unusual physical behavior when exposed to light or electric fields. The two most important phenomena are the reorientation of mesogens in electric fields and optical birefringence making them appealing for a number of applications.
Resulting from the anisotropy in the order of liquid crystals, the dielectric constant of an electric field differs when oscillating parallel or perpendicular to the director of the mesophases. Positive anisotropy is observed if the dielectric constant along the director is larger than in the direction perpendicular to it. If the dielectric displacement and the induced dipole moment are not parallel to the electric field the director experiences a force and for materials with a positive anisotropy the director will align parallel to the electric field. In contrast, mesogens with a negative anisotropy align perpendicular to an applied electric field.
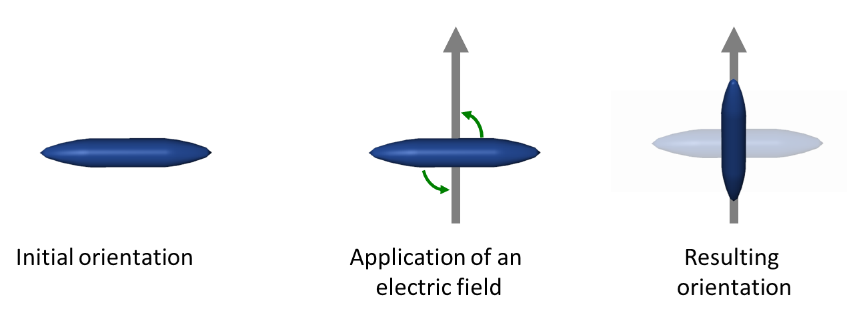
The anisotropy of liquid crystals leads to an optical property called birefringence. Birefringence is observed for materials having a refractive index depending of the propagation direction of light. For uniaxial materials a single direction governs the optical anisotropy (optical axis), while all directions perpendicular to it are optically equivalent. Rotating a sample around the optical axis does not affect the optical properties. Light with a polarization perpendicular to the optic axis is described by a refractive index no (for “ordinary”), while light whose polarization is in the direction of the optical axis is governed by the refractive index ne (for extraordinary). In isotropic media, both parts propagate with the same speed. In contrast, birefringent media will propagate the ordinary and the extraordinary waves with different speeds leading to a phase difference between both. The result is that light emerging from the birefringent medium is elliptically polarized. This behavior allows the characterization of mesophases by polarized optical microscopy under crossed polarizers.
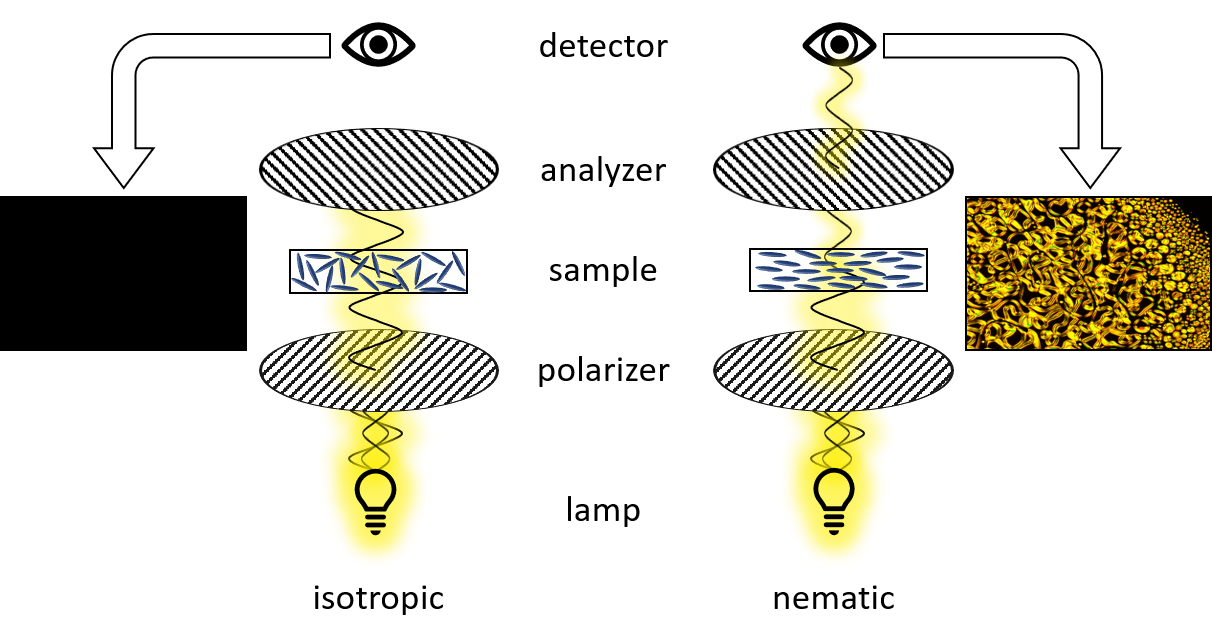
4. Characterization of Liquid Crystals
There is a variety of methods which are commonly employed to characterize liquid crystalline compounds. The most important methods will be described in the following.
Differential scanning calorimetry (DSC) is a thermoanalytic technique measuring the heat amount needed to increase the temperature of a sample compared to a reference. The reference chamber contains a sample with a well-defined heat capacity (such as water). The chambers are heated by separate heat sources to ensure their temperatures are always equal. The temperature of both chambers is constantly monitored and adjusted by a thermocouple. If a temperature difference is detected between the chambers, heat will be added to the cooler chamber to compensate for the difference.
DSC is commonly employed to determine the transition temperatures of liquid crystals. When a liquid crystalline sample undergoes a physical transformation more (endothermic process) or less (exothermic process) heat will be needed to maintain the same temperature between the reference and the sample chamber. If a solid melts for example to a liquid more heat is required to increase the temperature of the sample chamber at the same rate as the reference. The heat is absorbed by the sample as it undergoes the endothermic phase transition from solid to liquid. On cooling the sample will crystallize in an exothermic processes, thus less heat is required to raise the sample temperature. DSC is widely used to study subtle physical transformations such as glass transitions or mesophases.
DSC experiments result in a curve of heat flux versus temperature or time. Exothermic processes are usually shown with a positive peak, likewise a negative peak indicates an endothermic transition. The DSC curve allows for the determination of transition enthalpies by integrating the peak corresponding to a given transition. The enthalpy of transition is describe by the following equation:
K: Calorimetric Constant (depending on the instrument)
A: Area under the curve
Polarizing optical microscopy (POM) is an essential tool to characterize new liquid crystals. POM allows to identify transition temperature (like DSC) and additionally gives insight in the phase type. Therefore, a sample is heated under the microscope and the change in texture is observed when passing a phase transition. This textures and defect structure are characteristic for the phase type. However, the interpretation of these textures requires experience, especially for systems of higher order.
The setup of a polarized light microscope is similar to the setup of an optical microscope. It consists of a light source emitting white light through a lens and linear polarizer, which can be rotated by 360°. After being linearly polarized the light passes through the condenser, which bundles the light and assures homogeneous illumination of the sample. The sample is located on top of a hot stage. The transmitted light passes through the objective and a second polarizer – the analyzer. For studying liquid crystalline textures the analyzer is rotated by 90° in respect to the first polarizer (crossed polarizers). An isotropic medium like glass between the two polarizers now appears dark. However, as already mentioned before birefringent compounds interact with the polarized light and therefore appear bright under crossed polarizers. Finally the light reaches the ocular or a camera to capture the texture of the sample.
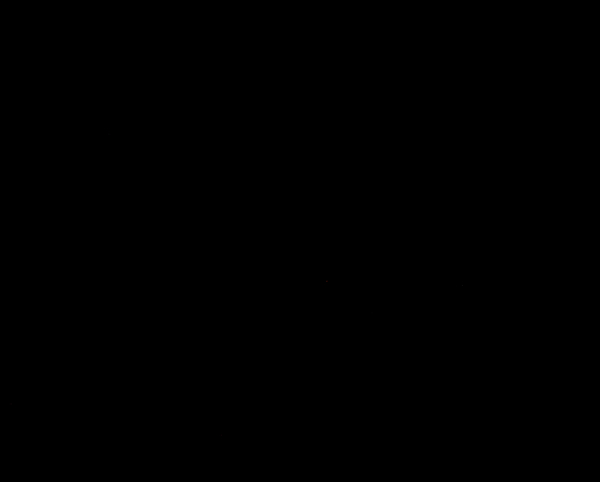
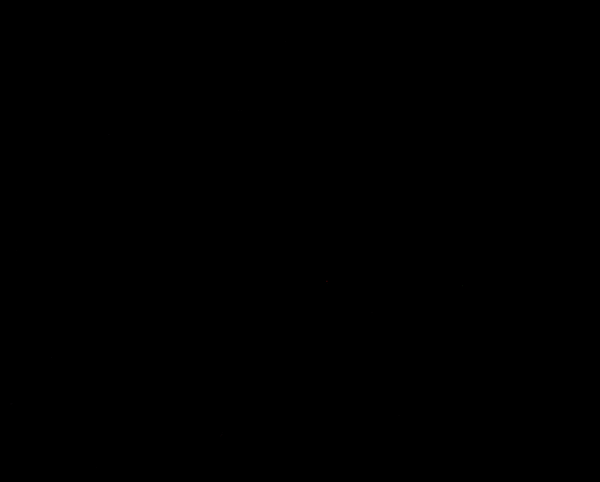
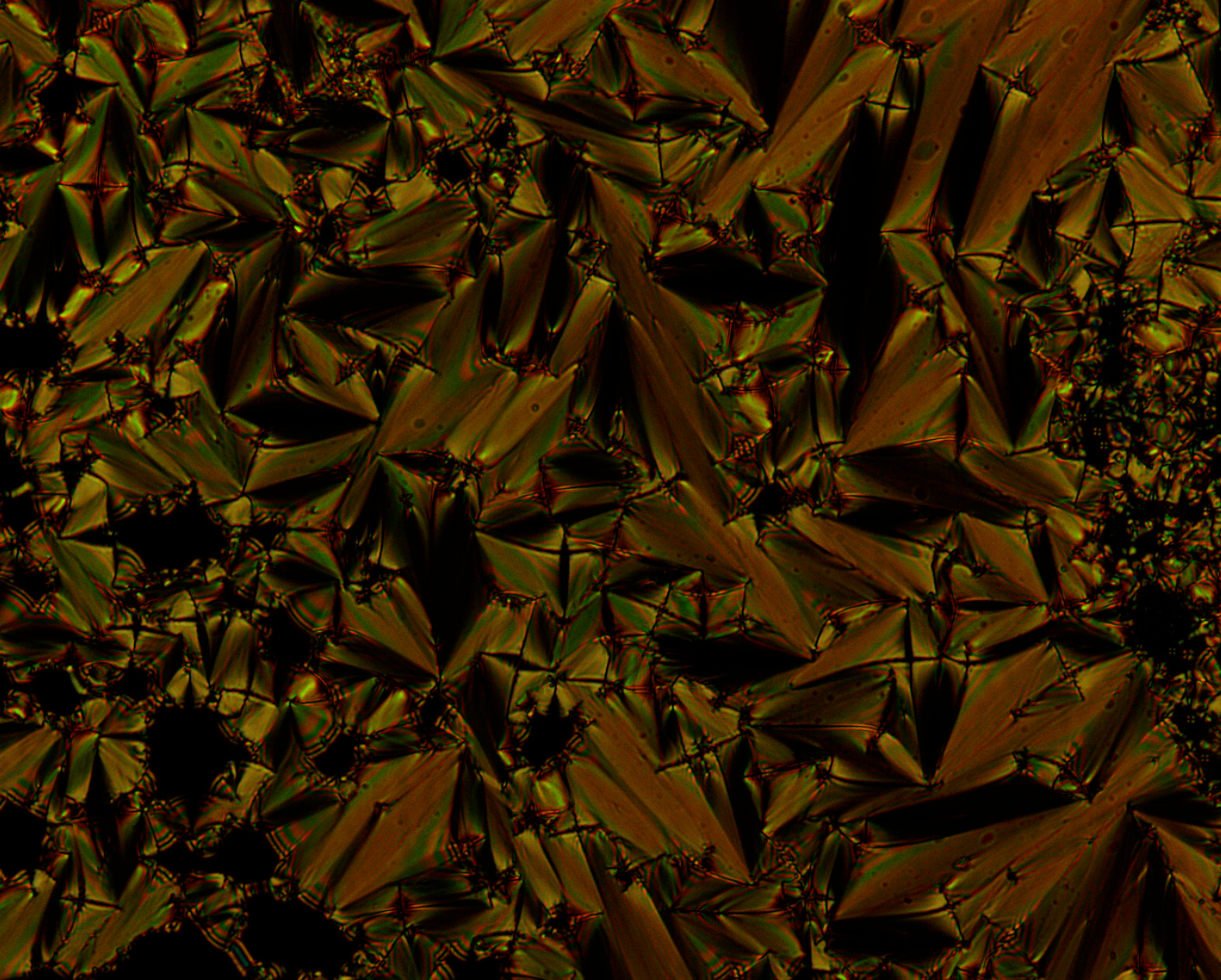
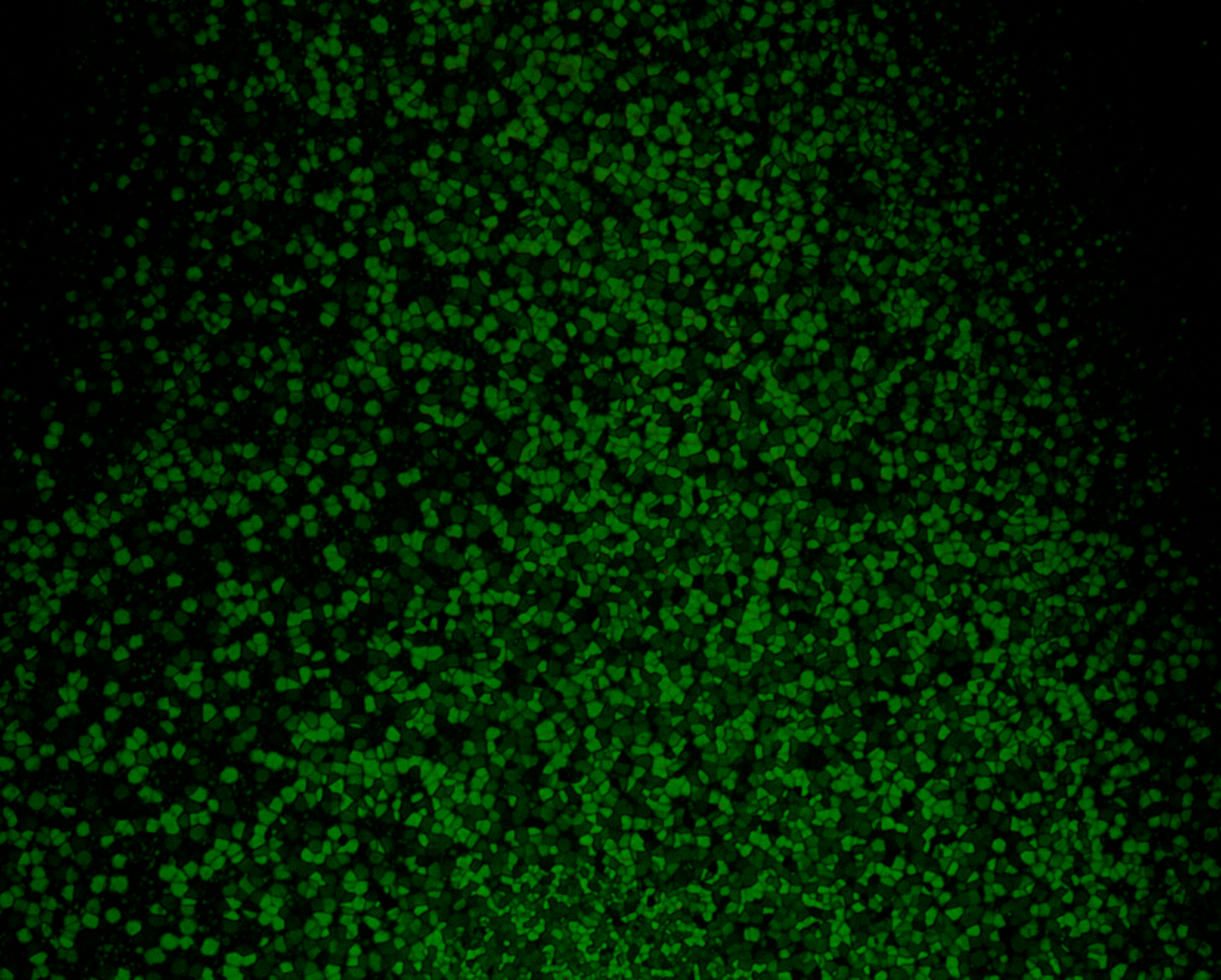
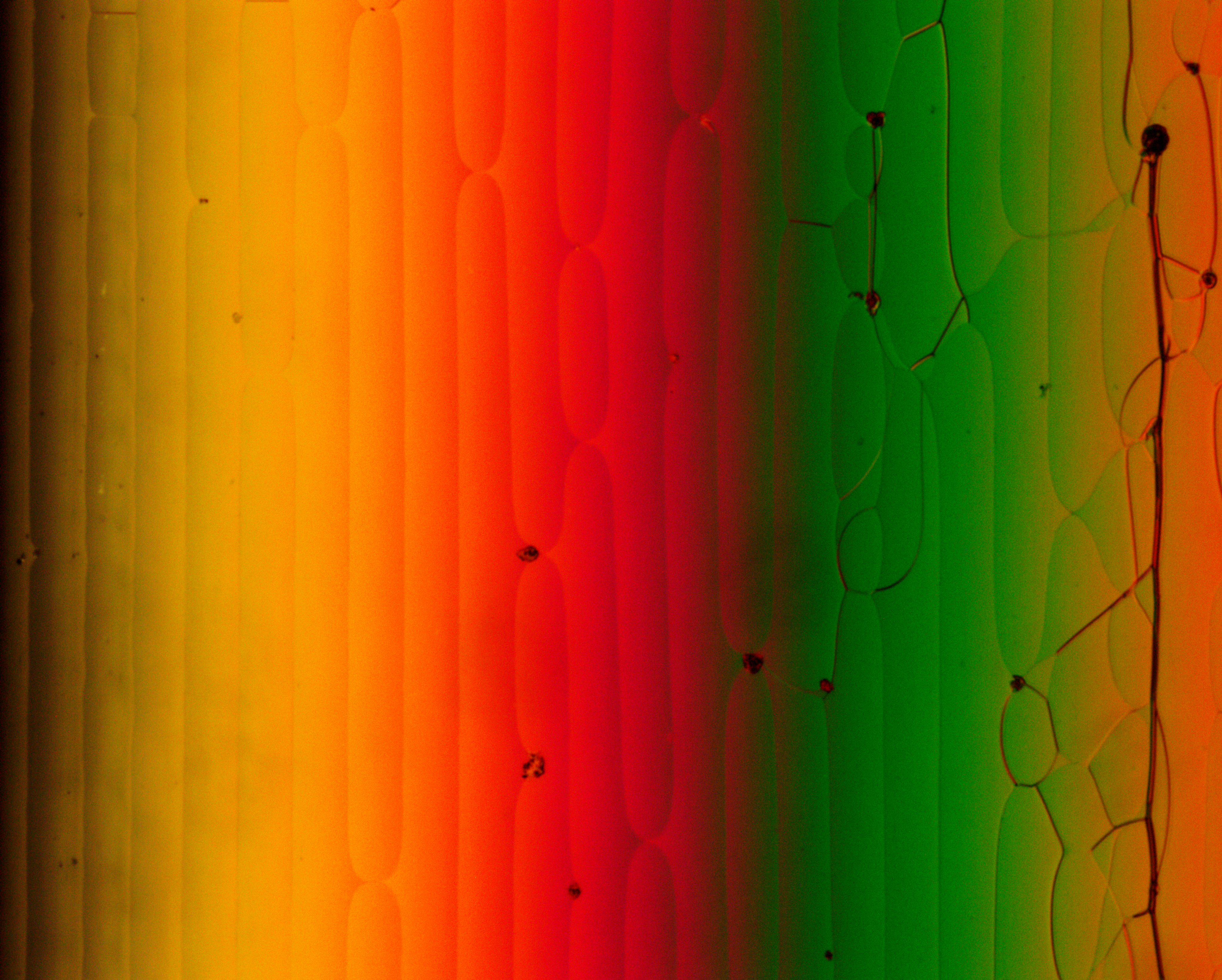
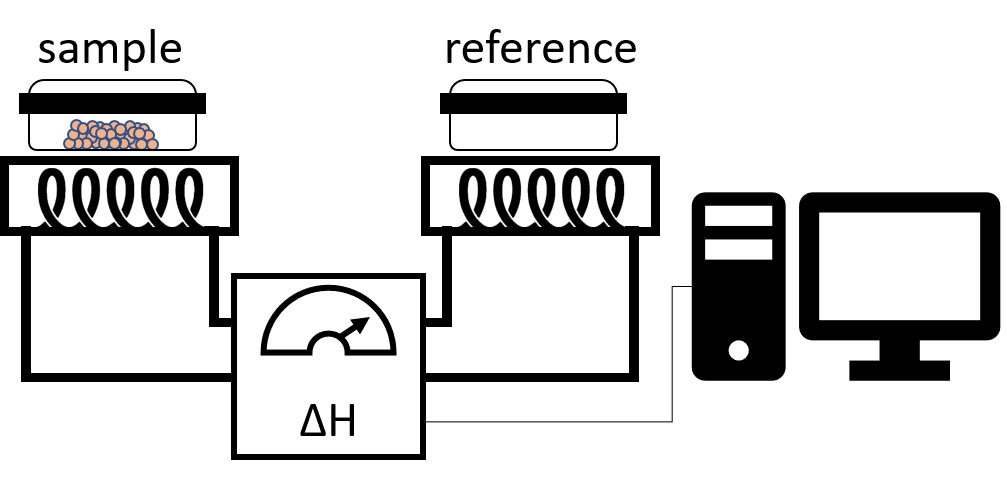
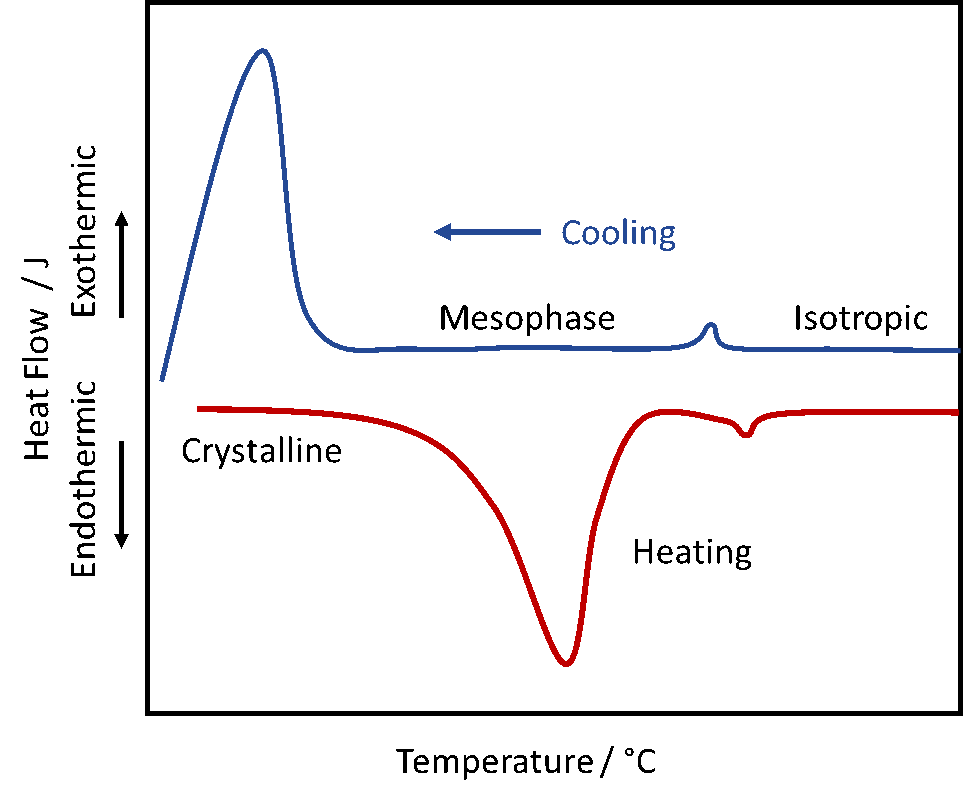
X-Ray diffraction (XRD) is a fundamental technique to analyze the atomic order of materials. It is based on the constructive interference of reflected X-rays when the path difference between adjacent planes of a crystals is an integer multiple of the wavelength of the X-rays. The mathematical description of this is called Bragg’s Law.
θ: angle between incident X-ray beam and scattering plane
λ: wavelength of the incident X-ray
The scattered X-rays form specific diffraction patterns depending on the order of the sample. While single crystals diffract rays into discrete directions, polycrystalline samples show a series of diffraction cones.
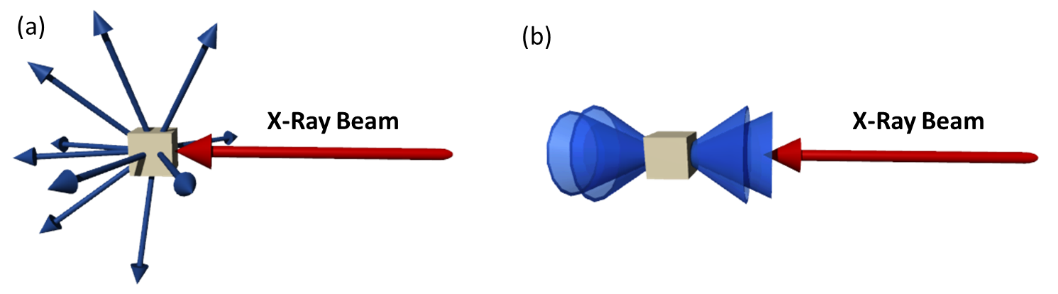
Two-dimensional (2D) XRD allows to collect and analyze X-ray diffraction pattern in two dimensions. A typical setup consists of an X-ray source (usually with a cupper or molybdenum source), a X-ray optics, which filters and focusses the X-ray beam and a goniometer, which rotates the sample in the X-ray beam. The scattered X-rays are detected by a 2d area detector. While data collection in 1D X-ray diffraction is limited to a single plane, 2D X-Ray diffraction is not yielding a large portion of additional diffraction rings. This allows to get a deeper understanding of the molecular arrangement and is useful to get insight in the molecular order of e.g. liquid crystals.
As already mentioned above, mesophases represent a state of matter with an intermediate order between an isotropic liquid and a solid. When heated to the isotropic state no long-range positional or orientational order is presence. The 2D diffraction pattern consists of a diffuse ring, which is caused by the short range positional order of a classical liquid. The radius of the ring represents the distance between the molecules along their width.
By cooling the sample down the mesophases is reached. A nematic phase has a long-range positional order but no positional order. When an unaligned sample diffracts X-rays the resulting diffraction pattern is similar to the isotropic state, but with sharper intensity distributions. In contrast, an aligned sample shows two sets of diffuse arcs. The radius of the larger ones represents the distance between the molecules along their widths.
Aligned smectic phases also show two sets of diffuse peaks in the diffraction pattern. The signal at small angles usually condense into sharp quasi-Bragg peaks, while the intensity distribution is not very sharp. This is attributed to the random arrangement of the molecules within the layers. In case of smectic C phases the angle between the layers normal and the director can be seen in the tilt between the diffraction signals at small and large angles.
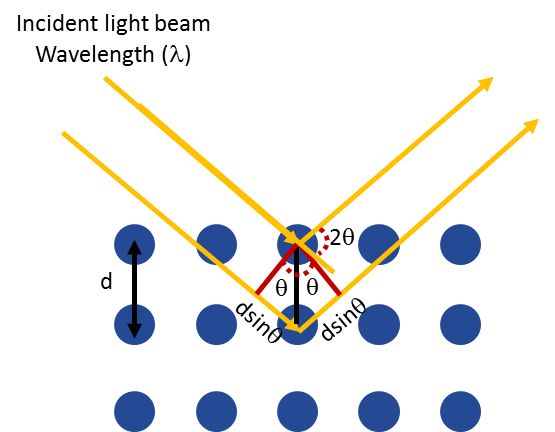