Research Highlights
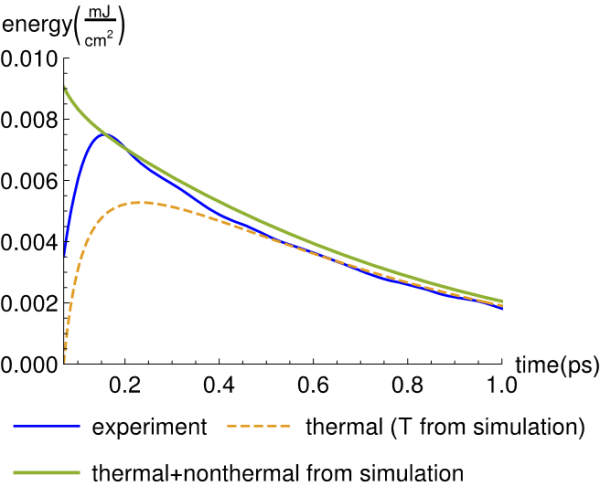
Relaxation of hot charge carriers: description developed beyond multi-temperature models
As known from numerous earlier experiments, two elementary processes must interact in the relaxation of hot charge carriers in metals, the electron-electron and the electron-phonon interaction. On the one hand, the electron system, considered as a whole, only loses energy through the scattering at the atoms and through the resulting stimulation of phonons; whereas the collisions between electrons alone, would keep the total electronic energy constant. On the other hand, it turns out that the electron-electron collisions have a strong influence on how the excited electrons are distributed over the energy bands. This, in turn, determines how effectively they can produce phonons, since the coupling of the electrons to the vibrations of the atoms depends strongly on which energy band the electron is currently in and with which type of phonon it can couple.
For these reasons, we need a theory of coupled rate equations that adequately accounts for both electron-electron scattering and electron-phonon scattering if we want to compare the results to the experiment. We recently carried out such a comparison for a particular material system, for a thin layer of lead on silicon. This material system was suitable because it can be produced in a very controlled way (the film consists of exactly 5 atomic layers), and because it is very well possible to follow experimentally the gradual energy loss of the electrons in the lead. Since the silicon has an energy gap that was larger than the quantum of energy of the light that was used, electrons in the silicon could not be excited in this experiment, nor could the electrons excited in the lead escape into the silicon. Therefore, this material system was very suitable for comparing experiment and theory. Both the strength of the electron-electron scattering and the electron-phonon coupling could be determined from a parameter-free calculation.
The figure shows the measured energy density in the experiment (green curve). Our novel rate equation theory (blue curve) reproduces the behaviour of the energy density, especially at short times (less than 300 femtoseconds after laser excitation), better than earlier, simpler theories that simply describe the hot electrons by a Fermi-distribution at a time-varying temperature (dashed curve).
Read more:
Peter Kratzer, Laurenz Rettig, Irina Yu. Sklyadneva, Evgueni V. Chulkov and Uwe Bovensiepen: “Relaxation of Photoexcited Hot Carriers Beyond Multitemperature Models: General Theory Description Verified by Experiments on Pb/Si(111)”
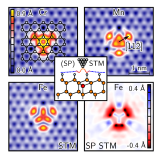
Atomic-scale detection of magnetic impurity interactions in bulk semiconductors
The identification of materials systems to be used as dilute magnetic semiconductors has so far been following a trial-and-error approach, resulting in slow progress of this field. For rational materials design, understanding the magnetic interactions in a dilute magnetic semiconductor on a fundamental, atomic level is a prerequisite. We promote spin-polarized scanning tunneling microscopy as a powerful method to extract atomic-scale information about the magnetic interactions of impurities in semiconductors.
The results of large-scale ab initio simulations put us in position to devise a generally applicable experimental strategy that exploits passivation of the dangling bonds at semiconductor surfaces in order to preserve the bulklike behavior of subsurface impurities. The latter is not the case in state-of-the-art cross-sectional scanning tunneling microscopy experiments, e.g., on Mn-doped GaAs.
In advantage over conventional spectroscopic techniques, such as electron paramagnetic resonance or x-ray magnetic circular dichroism, the proposed strategy provides a visual explanation why ferromagnetism is more difficult to achieve in Si than in GaAs.
Read more: Phys. Rev. B 92, 100407(R) (2015)
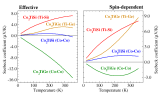
Interplay of growth mode and thermally induced spin accumulation in Al/Heusler alloy contacts
The feasibility of thermally driven spin injectors built from half-metallic Heusler alloys inserted between aluminium leads was investigated by means of ab initio calculations of the thermodynamic stability and electronic transport.
We have found that: (i) the interface between Al and the closely lattice-matched Heusler alloys of type Co2TiZ (Z=Si or Ge) is stable under various growth conditions; and (ii) the conventional and spin-dependent Seebeck coefficients in such heterojunctions exhibit a strong dependence on both the spacer and the atomic composition of the Al/Heusler interface, in other words, on whether a Ti-Z or a Co-Co plane makes the contact between Al and the Heusler alloy. Our results show that it is in principle possible to tailor the spincaloric effects by a targeted growth control of the samples.
Read more: Phys. Rev. B 89, 184422 (2014)
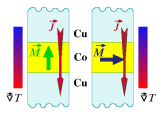
Large Seebeck magnetic anisotropy in thin Co films embedded in Cu determined by ab initio investigations
The longitudinal thermopower of a Cu/Co/Cu trilayer system exhibits an oscillatory dependence on the thickness of the Co layer, a behavior related to the formation of quantum well states in the minority spin channel. In addition, it is found to be very sensitive to a switching between an in-plane and out-of-plane magnetisation, a setup schematically shown in the enclosed figure.
The resulting magnetothermopower (MTP) is much larger than anticipated from the conventional anisotropic magnetoresistance (AMR). Our calculations, performed within the framework of spin-polarised relativistic Korringa-Kohn-Rostoker Green's function method combined with the Landauer-Buttiker conductance formula, establish a direct connection between the magnitude of the MTP signal and the asymmetry of the AMR around the Fermi energy. An enhancement of MTP based on this understanding may offer the possibility of implementing an efficient spin read-out thermoelectric device based on a single ferromagnetic layer.
Read more: Phys. Rev. B 88, 104425 (2013)