Reichenberger-Group
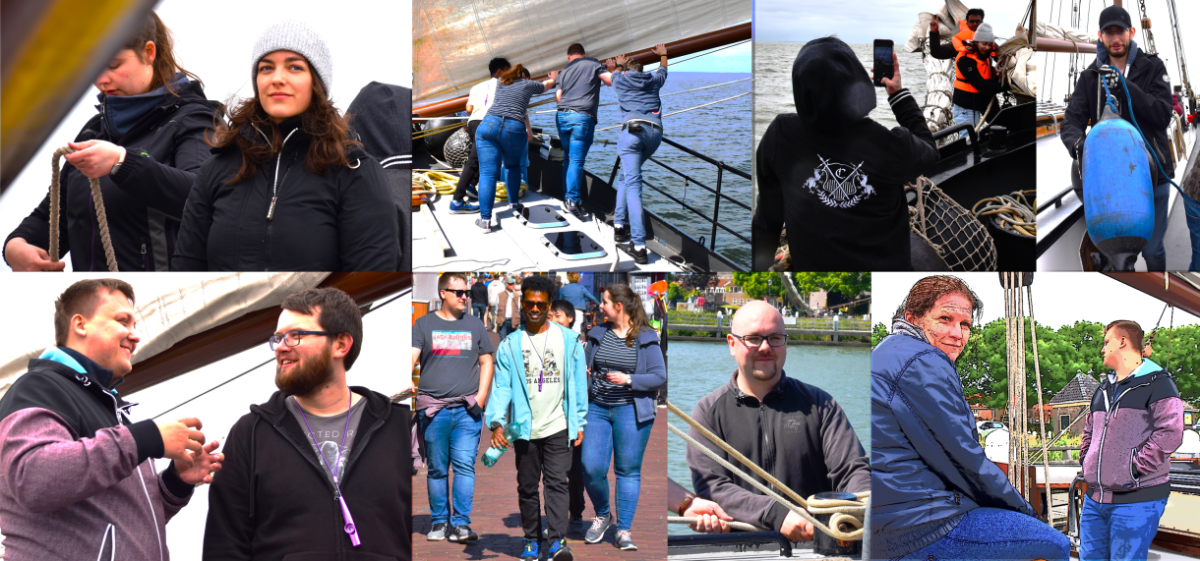
Welcome to the Reichenberger Group
Where laser-based nanoparticle processing meets catalysis research
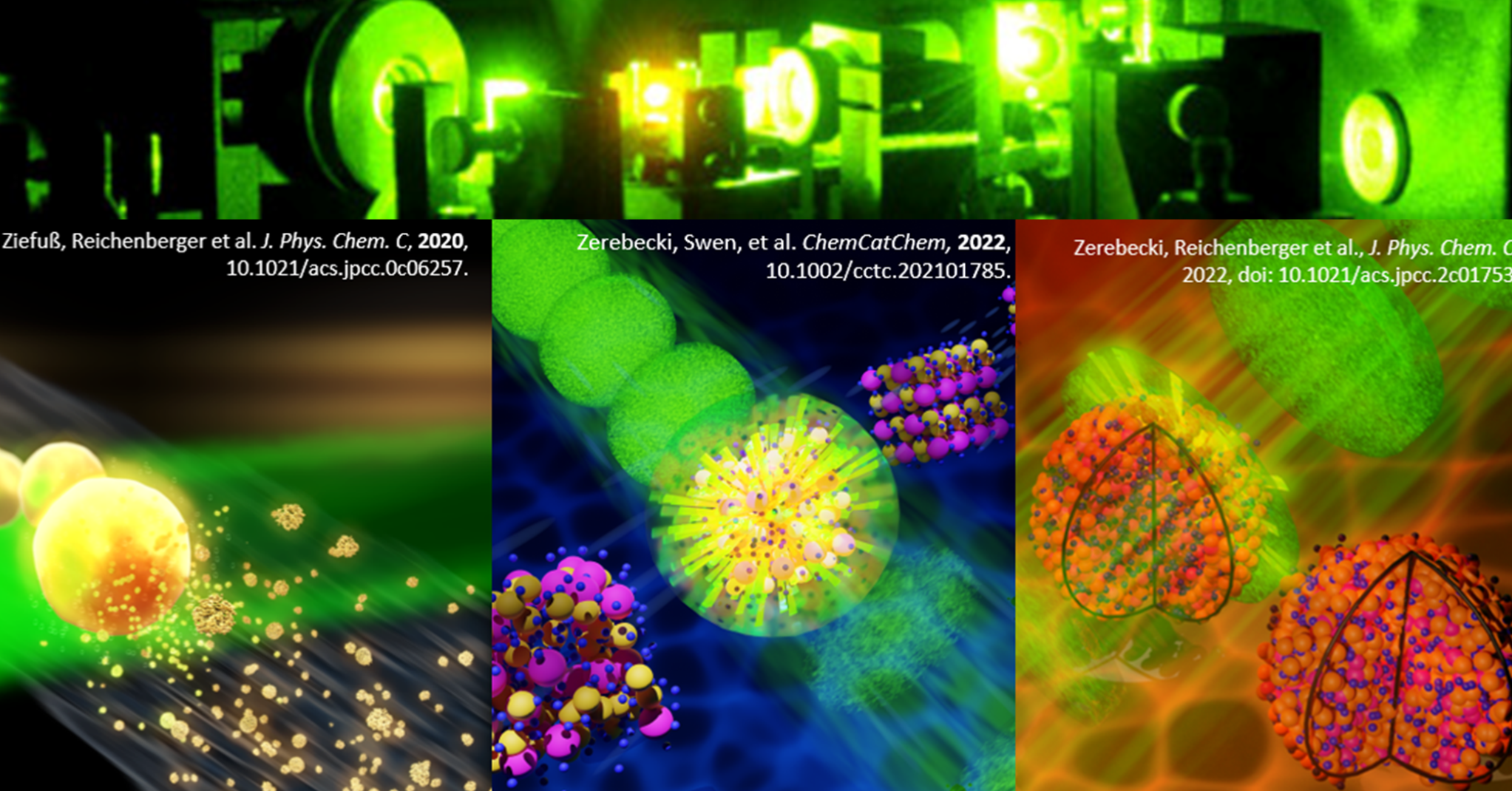
General research concept:
|
![]() |
The three pillars of my research
To achieve these ambitious goals, I follow three systematic paradigms:
- Mechanistic understanding of the role of disorder in material functionality, primarily focusing on (but not exclusive to) catalysis. Structural disorder is a natural occurrence and can be influenced by various factors, such as synthesis, processing, or material properties (e.g., size, composition, shape). This presents challenges in exploring disorder in nanoparticles. To investigate the role of structural disorder (e.g., surficial point or crystallographic defects in the volume), my group has developed a processing method that uses individual laser pulses to introduce defects or dopants into nanoparticles without altering their composition, size, or shape. The concept is (and has recently been) transferable to thin film structures.
- Transient structure changes when processing colloidal nanoparticles with single laser pulses:
The application of an intense laser pulse (e.g. >1010 W/m2) to nanoparticles triggers several subsequent electronic, heating-, and diffusion/phase transfer-related processes with partially overlapping timescales. My research hereby focuses on nanoparticles dispersed in liquid phase (far aim: supercritical fluids) beneficial for a fast cooling and structure conservation after laser-induced heating. To understand the transient laser-induced structure evolution occurring on hierarchical time scale ranging from ps (electronic effect), to ns (heating/diffusion/phase transfer) and further µs and beyond (ripening, further reactions with the surrounding liquid) my group employs static (TEM, XRD, XPS, Raman…) but also transient methods (currently transient SAXS, see DFG project below) to study and understand the structure evolution and dynamics nanoparticles undergo shortly after the laser-particle interaction (down to the first ps). Particular emphasis is given to the defect formation/annihilation and doping of nanomaterials (metals, oxides, alloys) following a given working hypothesis.
- Development of materials with enhanced activity, durability, and strategic material vectors:
Without a doubt, a successful energy transition requires both new technologies (Engineering) and improved materials (Chemistry). The respective materials vector is not only defined by the materials function itself but also external factors like political, health, and monetary aspects. Given the ephemerality of this material vector, particularly when considering the politically turbulent times we are in, a material synthesis strategy that can quickly react to the changing demands and constraints of the material vector is beneficial. In this regard, my group uses the laser-based synthesis and processing of nanoparticles which is scalable, currently providing productivities of nanoparticles up to several g/h and flexible, allowing the production of a material library of metals, alloys, metallic glasses, and oxides nanoparticles of comparable particle size. The synthesis is commonly conducted in liquids of free choice (including ionic liquids or supercritical fluids) whereby nanoparticles with high purity are gained without the need of surfactants. Given an electrostatic stabilization we commonly further quantitatively adsorb our synthesized nanoparticles to chosen support materials to e.g. gain a supported heterogenous catalyst. Apart from the laser-based material development, newer research activities of my group also address the mechanistic understanding of the adsorption processes with a particular focus on the adsorption kinetics and the facet selectivity.
.
I'm particularly (but not exclusively) interested groups that specialized in:
- selective oxidation catalysis looking for new materials (in particular defective or doped catalysts)
- electrocatalysis looking for complex alloy nanoparticle catalysts (including alloys with >5 elements)
- the quantification and characterization of point- and volume defects (EPR, Mössbauer, X-ray-based methods...)
- in situ or in operando methods requiring doped or defect-rich nanoparticle series (where size, shape are maintianed)
We constantly offer positions for B. Sc. and M. Sc. thesis.
For open PhD and Post-doc positions please visit: https://www.uni-due.de/stellenmarkt/
If the my research raised your interest and you are interested in collaborating, the following section summarizes out currently adressed material vector and used analysis methods:
Our Material Vector:
- Surfactant-free, defect-engineered, and/or doped nanoparticles for catalysis and energy research
- Pure nanoparticles made of user-defined material from noble metals to semiconductors, e.g. Pt, Pd, Ni, NiO, Cu, CuO, but also alloys made of FeMnNiCoCrMo and platin group metals, or metalic glases.
- Tailored heterogeneous catalysts: Supporting of laser-generated nanoparticles to almost any carrier material with a high mass loading, e.g. Carbon, Al2O3, ZrO2, TiO2, ZnO, …
Our Analytical Methods:
Available in our laboratories
- analytical disc centrifugation (ADC),
- Analytical Ultra Centrifugation,
- UV-Vis spectroscopy,
- Photoluminescence spectroscopy and Lifetime analytics
- Zeta potential measurement
- Cyclic voltammetry and impedance spectroscopy
- X-Ray fluorescence spectroscopy
- Gaschromatography
- XRD and FTIR (collaboration with other groups)
Advances methods accessible via ICAN
- XPS
- AES
- HR-TEM/EDX
- Raman